Introduction to MM Wave and THz
millimeter wave; terahertz; communication; imaging; radar; integrated circuit
Summary
This paper briefly introduces the research status of millimeter wave and terahertz technology, and summarizes some important development directions in the future according to the development trend at home and abroad. In terms of millimeter-wave technology, the research status and new progress of millimeter-wave chips in recent years are highlighted, and some hot millimeter-wave system applications are also introduced, such as millimeter-wave communication, millimeter-wave imaging, and millimeter-wave radar.
Compared with the millimeter wave frequency band, the utilization of terahertz spectrum is still in the exploratory stage. This paper focuses on some key technologies of terahertz, including terahertz source, terahertz transmission, terahertz detection, terahertz components, etc. Some applications of terahertz in astronomy, non-destructive testing, life science, safety, high-speed communication and other fields are briefly introduced.
1 Introduction
With the continuous exploration of the electromagnetic spectrum, human beings have gained a full understanding of electronics and optics, and through the research of electronics and optics, various devices have been developed, forming two relatively mature research and application technologies.
One is microwave and millimeter wave technology, which has been widely used in radar, radio astronomy, communication, imaging, navigation and other fields, and the other is optical technology, whose application has penetrated into all aspects of people’s daily life. However, between the millimeter wave and optical frequency bands, there are still abundant spectrum resources that have not been fully developed, that is, the terahertz frequency band. Traditionally, the microwave frequency band is defined as 300 MHz∼26.5 GHz, the millimeter wave frequency band as 26.5∼300 GHz, and the terahertz frequency band as 300∼10000 GHz (10 THz).
One of the more popular sayings now is that 0.3∼30 GHz is the microwave frequency band, 30∼300 GHz is the millimeter wave frequency band, and some people call 0.1∼10 THz the terahertz frequency band, as shown in Figure 1.
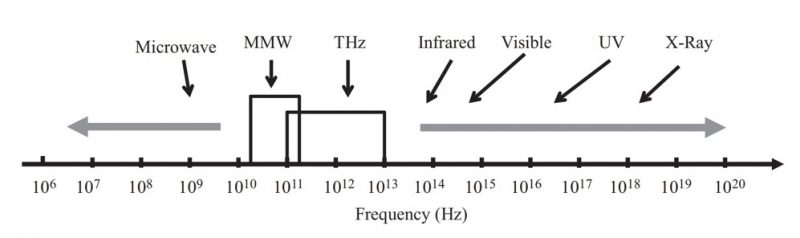
Figure 1 The location of millimeter and terahertz waves in electromagnetic spectrum
Due to the high cost of millimeter wave devices, they were mainly used in military applications before.
However, with the rapid development of high-speed broadband wireless communication, automotive assisted driving, security inspection, medical detection and other application fields, millimeter waves have also been widely researched and applied in civilian fields in recent years. At present, in the golden communication frequency band below 6 GHz, it is difficult to obtain a wider continuous spectrum, which seriously restricts the development of the communication industry.
In contrast, there are still a large number of potential underutilized spectrum resources in the mmWave frequency band. Therefore, millimeter wave has become a research hotspot in the 5th generation mobile communication.
In 2015, at the WRC2015 conference, the candidate frequency bands for 5G mobile communication research were determined: 24.25∼27.5 GHz, 37∼40.5 GHz, 42.5∼43.5 GHz, 45.5∼47 GHz, 47.2∼50.2 GHz, 50.4∼52.6 GHz, 66∼ 76 GHz and 81∼86 GHz, of which 31.8∼33.4 GHz, 40.5∼42.5 GHz and 47∼47.2 GHz are allowed as additional frequency bands under certain conditions of use. Various millimeter-wave devices, chips, and applications are being developed in full swing.
Compared with microwave frequency bands, millimeter-wave has its own characteristics. First, millimeter wave has a shorter operating wavelength, which can effectively reduce the size of devices and systems; second, millimeter wave has abundant spectrum resources, which can meet the needs of future ultra-high-speed communication.
In addition, due to the short wavelength, millimeter waves have higher resolution in radar, imaging, etc.
So far, people have carried out a lot of research on millimeter wave, and various millimeter wave systems have been widely used. With the rapid development of civilian technologies such as the 5th generation of mobile communications, auto-driving, and security checks, millimeter waves will be widely used in all aspects of people’s daily lives.
Terahertz research mainly focuses on the 0.1∼10 THz frequency band. This is a very wide and special spectral region.
At first, this frequency band was called “THz Gap” because it was sandwiched between two relatively mature spectrums, namely, the electronic spectrum and the optical spectrum. Its low frequency band overlaps with the millimeter-wave frequency band in the electronics field, and its high frequency band overlaps with the far-infrared frequency band (wavelength 0.03∼1.0 mm) in the optical field.
Due to the particularity of this field, a blank area of early research was formed.
However, with the development of research, the importance of terahertz spectrum and technology to physics, chemistry, biology, electronics, radio astronomy and other fields has gradually emerged, and its application has also begun to penetrate into many aspects of social economy and national security, such as biological imaging, THz spectrum rapid detection, high-speed communication, through-wall radar, etc.
The reason why terahertz has good application prospects is mainly due to its spectral resolution, safety, perspective, transient and broadband characteristics.
For example: the vibration and rotation frequencies of many biological macromolecules in nature are in the terahertz frequency band, which provides an effective means for detecting biological information; the photon energy in the terahertz frequency band is low, it will not cause damage to the detection body, and non-destructive testing can be realized; Terahertz waves have a good penetrating ability to dielectric materials, so they can be used as a means of detecting hidden objects; the typical pulse width of a terahertz pulse is on the order of picoseconds, and a terahertz time-domain spectrum with a high signal-to-noise ratio can be obtained, which is easy to perform spectral analysis on various materials; in addition, the bandwidth of the terahertz frequency band is very wide, ranging from 0.1∼10 THz, which can provide abundant spectrum resources for ultra-high-speed communication.
This paper mainly focuses on the development of the millimeter wave and terahertz fields in recent years, and summarizes the millimeter wave and terahertz technologies and applications respectively.
In terms of millimeter-wave technology, the development of millimeter-wave chips is mainly introduced in combination with some popular millimeter-wave frequency band system applications, such as millimeter-wave communication, millimeter-wave imaging, and millimeter-wave radar.
In terms of terahertz technology, the research progress of terahertz wave generation technology, terahertz wave transmission technology and terahertz wave detection technology is introduced, and the typical applications in the field of terahertz are given correspondingly while the key components are introduced. The introduction mainly includes terahertz biological applications, medical applications, imaging applications, atmospheric science, environmental science, communication technology and national security, etc.
Finally, the future development of the millimeter-wave and terahertz fields is prospected, and some directions worth focusing on in the future are pointed out.
2 millimeter wave technology
In recent decades, millimeter wave technology has made great progress. This section will introduce the progress of some millimeter-wave key devices, key technologies and systems.
2.1 millimeter wave chip
Traditional millimeter-wave monolithic integrated circuits mainly use compound semiconductor technology, such as gallium arsenide (GaAs), indium phosphide (InP), etc., which have good performance in the millimeter-wave frequency band and are the mainstream integrated circuit technology in this frequency band.
On the other hand, silicon-based (CMOS, SiGe, etc.) millimeter-wave and submillimeter-wave integrated circuits have also made great progress in the past decade. In addition, high-power high-frequency devices based on gallium nitride (GaN) technology are also rapidly expanding to the millimeter-wave frequency band. The following will introduce them separately.
2.1.1 GaAs and InP millimeter wave chips
In the past ten years, GaAs and InP processes and devices have made great progress. The millimeter-wave device types based on this type of process mainly include high electron mobility transistors (HEMTs), modified high electron mobility transistors (mHEMTs), and heterojunction bipolar transistors (HBTs).
At present, the cut-off frequency (ft) of GaAs mHEMT, InP HEMT and InP HBT all exceeds 500 GHz, and the maximum oscillation frequency (fmax) exceeds 1 THz.
In 2015, the Northrop Grumman Company of the United States reported the InP HEMT amplifier working at 0.85 THz. In 2013, the Teledyne Company of the United States and the Jet Propulsion Laboratory of the California Institute of Technology reported the InP HEMT amplifier working at 0.67 THz. In 2012 and 2014, the Fraunhofer Institute for Applied Solid State Physics in Germany reported mHEMT amplifiers with operating frequencies exceeding 0.6 THz.
2.1.2 GaN millimeter wave chip
As a third-generation wide bandgap compound semiconductor, GaN has the advantages of large bandgap width, high electron mobility and breakdown field strength. The power density of the device is more than 5 times that of GaAs, which can significantly increase the output power and reduce the volume and cost. With the gradual maturity of GaN material preparation technology in the 1990s, GaN devices and circuits have become a hot spot in the field of compound semiconductor circuit development
The United States, Japan, Europe and other countries regard GaN as the development focus of microwave and millimeter wave devices and circuits.
In the past ten years, silicon carbide (SiC), a low-cost substrate material for GaN, has also gradually matured. Its lattice structure matches GaN, and its thermal conductivity is good, which greatly accelerates the development of GaN devices and circuits.
In recent years, GaN power devices have developed rapidly in the field of millimeter waves. Eudyna Corporation of Japan reported a device with a gate length of 0.15 µm, and the power output density reached 13.7 W/mm at 30 GHz. HRL of the United States reported a variety of E-band, W-band and G-band GaN-based devices, the W-band power density exceeds 2 W/mm, and the power density reaches 296 mW/mm at 180 GHz.
Domestic GaN power devices in the microwave frequency band have basically matured, and GaN power devices in the W band have also made progress. The Ka-band GaN power MMIC developed by the Nanjing Institute of Electronic Devices has a pulse output power of 15W in the 34∼36 GHz frequency band, an additional efficiency of 30%, and a power gain of more than 20 dB.
2.1.3 Silicon-based millimeter-wave chips
Silicon-based technology has traditionally been dominated by digital circuit applications. With the continuous development of deep submicron and nanometer technology, the feature size of silicon-based technology is continuously reduced, and the shortening of gate length makes up for the lack of electron mobility, so that the cut-off frequency and maximum oscillation frequency of transistors continue to increase.
This makes it possible to apply silicon technology in the millimeter wave or even terahertz frequency band. The International Technology Roadmap for Semiconductors predicts that by 2030 the feature size of the CMOS process will be reduced to 5 nm, while the cutoff frequency ft will exceed 700 GHz.
The cut-off frequency ft and the maximum oscillation frequency fmax of the SiGe process transistors of the German IHP Institute have reached 300 GHz and 500 GHz respectively, and the corresponding operating frequency of silicon-based process circuits can be extended to more than 200 GHz.
Due to the huge advantages of silicon technology in terms of cost and integration, the research on silicon-based millimeter-wave and submillimeter-wave integrated circuits has become one of the current research hotspots.
The University of Florida designed a 410 GHz CMOS oscillator;
The University of Toronto in Canada has developed a 170 GHz amplifier based on SiGe HBT process, a 160 GHz mixer and a 140 GHz frequency converter based on CMOS process;
The University of California, Santa Barbara, etc. have developed a 150 GHz amplifier based on a CMOS process, etc.;
Cornell University in the United States developed a 480 GHz frequency multiplier based on CMOS technology.
In terms of system integration, the University of Toronto designed a 140 GHz CMOS receiver chip and a 165 GHz SiGe on-chip transceiver system, and the University of California, Berkeley, for the first time integrated a 60 GHz band silicon-based analog transceiver circuit with a digital baseband processing circuit On the CMOS chip, the Singapore Institute of Microelectronics has also realized a 60 GHz CMOS transceiver chip including an on-chip antenna. The University of California, Los Angeles reported a 0.54 THz frequency synthesizer, and the German Wuppertal University developed a 820 GHz Silicon-based SiGe active imaging system, the University of California, Berkeley successfully developed a 380 GHz radar system using SiGe technology.
Japan’s NICT and others have implemented 300 GHz transceiver chips based on CMOS technology and achieved a transmission rate of more than 10 Gbps, but the transmission distance is very short due to the absence of power amplification and low-noise circuits. By adopting silicon-based technology, all circuits including digital circuits can be integrated on a single chip, so it is expected to greatly reduce the cost of mmWave communication systems.
In terms of millimeter-wave and submillimeter-wave silicon-based integrated circuits, mainland China started a little late, but with the support of the national “973” plan, “863” plan and the Natural Science Foundation of China, research has been carried out rapidly and progress has been made.
The State Key Laboratory of Millimeter Waves of Southeast University has successfully designed devices such as Q, V and W band amplifiers, mixers, VCOs, W-band receivers, Q-band multi-channel transceivers, etc. based on 90 nmCMOS technology, as well as 200 GHz CMOS frequency multiplier and SiGe oscillator to 520 GHz, etc.
2.2 millimeter wave electric vacuum device
Millimeter wave integrated circuits have many advantages such as small size and low cost, but the power is limited.
In order to obtain higher output power, electric vacuum devices can be used, such as the klystron (Klystron) developed by the Canadian CPI company, which has obtained a pulse output power of more than 2000 W in the W band, and the traveling wave tube developed by the Beijing Institute of Vacuum Electronics. The pulse output power of the (TWT) amplifier in the W-band exceeds 100 W. The University of Electronic Science and Technology of China has also successfully designed a TWT power amplifier in the W-band. The Gyrotron developed by the Hefei Institute of Physical Science, Chinese Academy of Sciences has achieved The pulse output power of 0.9 MW is achieved, which is comparable to the foreign level.
2.3 mmWave applications
In recent years, the continuous improvement of the performance of millimeter-wave devices and the continuous reduction of cost have greatly promoted the application of millimeter-wave in various fields.
At present, the applications based on the millimeter-wave frequency band are mainly reflected in millimeter-wave communication, millimeter-wave imaging, and millimeter-wave radar.
2.3.1 Millimeter wave communication
With the rapid development of wireless communication technology, the spectrum of the golden communication frequency band below 6 GHz is already very crowded, and it is difficult to meet the needs of future wireless high-speed communication. However, on the contrary, in the mmWave frequency band, the spectrum resources are abundant but still not fully developed and utilized.
In terms of mobile communication, the literature explores the millimeter wave mobile communication system scenarios, network structure and air interface. In the current 5th generation mobile communication (5G) research, several millimeter wave frequency bands have become 5G candidate frequency bands. Millimeter wave technology will play a pivotal role in the development of 5G. In short-distance high-speed communication systems, the 60 GHz frequency band has been widely studied and applied.
Europe, the United States, Canada, South Korea, Japan, Australia, and my country have successively opened up free spectrum resources in this frequency band. The 60 GHz frequency band is at the peak of atmospheric attenuation. Although it is not suitable for long-distance communication, it can be used for short-distance transmission without causing too much interference to the surroundings.
In recent years, various systems and standards such as high-speed Gbps communication, WirelessHD, WiGig, near field communication, IEEE 802.11ad, IEEE802.15.3c have been developed in the 60GHz frequency band.
The Southeast University in China proposed the ultra-high-speed short-range wireless transmission standard (Q-LINKPAN) working in the 45 GHz frequency band, and its short-range part has become the IEEE 802.11aj international standard.
The atmospheric attenuation of the 45 GHz frequency band is less than 1 dB/km, so it can not only achieve high-speed short-distance transmission like the 60 GHz frequency band, but also is suitable for long-distance transmission. At present, the experimental system has achieved a transmission rate of 2 Gbps at a transmission distance of 82 m , and developed a corresponding millimeter-wave chip that supports Gbps transmission.
Satellite communication has a wide coverage and is an important means to ensure remote areas and maritime communications and emergency communications. At present, its working frequency bands are mainly concentrated in the L, S, C, Ku and Ka bands. With the deepening of satellite communication research, attempts have been made to higher frequency bands. Because the millimeter wave frequency band can provide wider bandwidth, it can achieve higher communication rate.
In addition, low power consumption, small size, anti-interference, and high spatial resolution are all worthy of use.
At present, the main research direction of satellite and ground communication is concentrated on two windows with small atmospheric attenuation, Q band and W band, and the 60 GHz band is considered to be an important frequency band for inter-satellite communication. In addition, millimeter-wave radio-on-fiber (RoF) systems have also been rapidly developed. Optical fibers have the advantages of low cost, large channel bandwidth, low loss, and strong anti-interference ability, and have become an indispensable part of modern communication systems.
As mentioned above, millimeter waves have the advantages of large transmission capacity and small size, but also have the disadvantages of large space transmission loss. The millimeter-wave RoF system combines the advantages of millimeter-wave and optical fiber communication, and is an effective means to realize long-distance transmission of broadband millimeter-wave communication. Since the concept of wireless communication over light was proposed in 1990, this field has become a research hotspot in the millimeter-wave frequency band. Many research groups have conducted research in different millimeter-wave frequency bands, such as 60 GHz, 75∼110 GHz, 120 GHz, 220 GHz, 250 GHz, etc.
2.3.2 Millimeter wave imaging
Utilizing the advantages of millimeter wave penetration and safety, millimeter wave imaging can effectively image the detected object, and has been widely studied in national security, airport security inspection, atmospheric remote sensing, etc. According to the imaging mechanism, it can be divided into passive imaging and active imaging.
The millimeter-wave passive imaging is to realize the imaging by detecting the radiation energy of the measured object itself and distinguishing the difference in the radiation intensity of different substances.
From the point of view of mechanism, passive imaging is a safe imaging method, which will not cause electromagnetic interference to the environment, but has higher requirements on the strength of the signal itself and the sensitivity of the receiver. A lot of research has been carried out on millimeter wave passive imaging technology at home and abroad.
The millimeter-wave active imaging mainly transmits a millimeter-wave signal of a certain intensity through the millimeter-wave source, and detects the difference between the measured object and the environment by receiving the reflected wave of the measured object, and then performs inversion imaging.
The active imaging system can detect non-metallic objects including plastics, which is less affected by the environment, obtains a large amount of information, and can effectively perform three-dimensional imaging.
Commonly used active imaging systems mainly include focal plane imaging and synthetic aperture imaging. The millimeter wave imaging system has been applied to the security check of many airports at home and abroad.
Sun Xiaowei’s team at the Shanghai Institute of Microsystems has successfully developed a millimeter-wave imaging security inspection system, and Fan Yong’s team at the University of Electronic Science and Technology of China has successfully developed a millimeter-wave dynamic imaging system.
2.3.3 Millimeter wave radar
Millimeter wave radar has the characteristics of wide frequency band, short wavelength, narrow beam, small size, low power consumption and strong penetration.
Compared with laser infrared detection, its strong penetrating characteristics can ensure that the radar can work in foggy, rainy, snowy and dusty environments, and is less affected by the weather. Compared with the radar in the microwave band, the use of the short wavelength of the millimeter wave can effectively reduce the volume and weight of the system and improve the resolution.
These characteristics make millimeter-wave radar have important applications in automobile collision avoidance, helicopter obstacle avoidance, cloud detection, missile guidance, etc. Microwave and millimeter wave automotive anti-collision radars are mainly concentrated in the 24 GHz and 77 GHz frequency bands, which are one of the core technologies for future intelligent driving or automatic driving. In the research of helicopter millimeter-wave anti-collision radar, people pay special attention to the detection effect of millimeter-wave radar on power lines and so on.
Millimeter waves also have important applications in atmospheric remote sensing, among which millimeter wave cloud radar is representative. The millimeter-wave cloud radar is mainly used to detect precipitation clouds, and is used to detect the macroscopic and microscopic parameters inside the cloud, reflecting the thermal and dynamic processes of the atmosphere.
Due to the short wavelength of the millimeter wave, it shows high measurement accuracy and resolution in cloud detection, and has the advantages of penetrating thick clouds with more water content. Ge Junxiang’s team at Nanjing University of Information Science and Technology has developed a W-band cloud radar, and Lu Xin’s team at Beijing Institute of Technology is developing a 94/340 GHz dual-band cloud radar. In addition to civilian use, millimeter-wave radar also has very important applications in the military. For example, in precision-guided weapons, millimeter-wave radar guidance is a core technology and an effective means of carrying out precise strikes on targets around the clock.
3 Terahertz Technology
Compared with millimeter-wave technology, the research on terahertz technology is still in the exploratory stage. This section mainly introduces some key technologies in the terahertz field and some current popular applications.
Terahertz technology mainly includes terahertz wave source, terahertz transmission and terahertz detection, etc., and its key components can be divided into passive components and active devices.
Passive components include terahertz transmission lines, filters, couplers, antennas, etc., while active components include terahertz mixers, frequency multipliers, detectors, amplifiers, oscillators, etc.
3.1 Terahertz source
With the development of terahertz wave generation technology, many valuable new progresses have been made in the research of terahertz sources. The development of low-cost, high-power, room-temperature stable terahertz sources is the basis for the development of terahertz technology. There are various classifications of terahertz sources, and according to the generation mechanism, they can be divided into those based on optical effects and those based on electronics.
According to the source type, it can be divided into three categories: incoherent thermal radiation source, broadband THz radiation source, and narrowband THz continuous wave source.
3.1.1 Incoherent thermal radiation sources
The incoherent thermal radiation source converts thermal energy into light energy in thermal equilibrium, producing a continuous spectrum.
Prime examples are the sun in everyday life, and incandescent lamps. Due to the low power of the terahertz wave generated by it, the application prospect is relatively limited.
3.1.2 Broadband THz radiation source
Broadband terahertz radiation sources are currently mainly used in spectroscopic systems, which are mainly generated by pulses with a period of tens to hundreds of femtoseconds, and the spectrum contains ultra-broad spectrum components up to tens of terahertz.
Generation methods include:
- Photoconductive antenna:
The main mechanism of the terahertz radiation of the photoconductive antenna is that the photoconductive antenna generates carriers under the irradiation of light pulses, accelerates the movement under the action of the electric field, generates a transient current on the surface, and then radiates terahertz electromagnetic waves. output energy.
In recent years, many studies have been carried out at home and abroad on the generation of broadband terahertz waves by photoconductive antennas. - Light rectification method:
The optical rectification method uses the nonlinear optical rectification effect to cause two beams or a high-intensity monochromatic beam to generate difference frequency or sum frequency oscillation when propagating in the medium. It is characterized in that it can achieve terahertz ultra-wideband output, but the output The energy is relatively low. Based on this principle, terahertz radiation sources have been greatly developed. - Air plasma method:
The principle of the air plasma method is to use laser focus to break down the air to generate terahertz radiation. - Semiconductor surface: The basic working principle of the terahertz radiation source based on the semiconductor surface can be summarized as the surface electric field effect and the photogenerated Temper effect.
For some wide-bandgap semiconductor materials, there are surface states on the surface, and the surface electric field will be formed due to the inconsistency of the Fermi energy level between the surface and the interior.
Under the action of this electric field, the carriers excited by the laser will form a transient current, thus forming terahertz radiation.
For some narrow-bandgap semiconductor materials, due to their large absorption coefficient, a large number of carriers will be formed on the surface of the semiconductor, and electrons and holes will separate the positive and negative charges in space when they diffuse into the semiconductor, forming a photogenerated tempepe Electric field, radiating terahertz waves.
The feature of this method is that it is simple and easy to operate, but the radiation power is low.
3.1.3 Narrowband terahertz continuous wave source
The goal of narrow-band terahertz radiation sources is to generate continuous terahertz waves with narrow linewidths.
Commonly used methods include:
- Using electronic devices to design oscillators, especially based on submillimeter wave oscillators, to increase the operating frequency of the oscillators, so as to design and implement oscillators suitable for the THz frequency band.
Due to this feature, the operating frequencies of the reported terahertz sources are mainly concentrated in the lower terahertz frequency band.
However, on this basis, terahertz waves with a frequency of about 1 THz or even higher have been obtained by using frequency multiplication chains. - Terahertz quantum cascade laser (THz-QCL), as a kind of coherent light source, realizes population inversion based on transition between conduction band and subband electronic energy states and phonon resonance-assisted tunneling.
With the rapid development of quantum cascade lasers, it can be used to study the motion of matter at a microscale, such as electron microscopic transport, nanophotonics, etc.
At the same time, due to its compact structure, it has high application value in many fields, such as astrophysics and atmospheric science, space communication, precision spectral measurement, security inspection field and terahertz imaging, etc. - The free electron laser converts the kinetic energy of the relativistic electron beam moving in the magnetic field into photon energy, thereby generating laser light, which is characterized by high energy and high coherence.
Due to its continuity, the radiation wavelength can be tuned to any wavelength, which is very suitable for use as a terahertz radiation source, but the disadvantages of free electron lasers are high power consumption, large size and high cost, so free electron lasers are basically used in laboratory environments. - Optically pumped terahertz laser: The terahertz frequency band corresponds to the rotational energy levels of many polar molecules, and the optically pumped terahertz laser inverts the number of particles between the rotational energy levels of these polar molecules, thereby generating terahertz radiation.
In related work at home and abroad, commonly used gases are CH3F, NH3, D2O, CH3OH, etc. - Difference frequency terahertz radiation source: The difference frequency terahertz radiation source mainly uses the difference frequency effect of nonlinear crystals to generate coherent narrowband terahertz radiation.
In this method, two laser beams with different wavelengths, that is, different frequencies, are required to pump nonlinear crystals at a certain angle, such as GaSe, ZnGeP2, GaAs, GaP, LiNbO3, and organic crystal DAST.
The frequency of the terahertz wave depends on the wavelength of the pump light, which can be easily tuned. - Optical parametric method: The optical parametric method uses a beam of pump light to enter the crystal to excite Stokes light and electromagnetic couplers.
Under the combined action of pump light and Stokes light, the electromagnetic couplants undergo stimulated Raman scattering, realizing terahertz radiation
3.2 Terahertz Transmission
Due to the large loss of terahertz waves in the air, its transmission structure is an indispensable part.
The research on the loss and dispersion characteristics of different transmission structures has gradually become a research hotspot in the field of terahertz. Researchers from all over the world are trying to find terahertz transmission structures with low loss, low dispersion, and high power capacity, that is, to find materials and structures suitable for transmitting terahertz waves.
As far as the research method is concerned, it is mainly based on the fact that the terahertz frequency band is sandwiched between the millimeter wave frequency band and the optical frequency band in the wave spectrum. People try to improve the mature transmission materials in these frequency bands and apply them to the terahertz frequency band. These attempts include Metal circular waveguides, parallel plane metal waveguides, metal wire waveguides, dielectric waveguides with metal coatings, all-dielectric waveguides, subwavelength periodic hole arrays, microstructured optical fibers with elliptical hollow fiber cladding, two-wire transmission structures, photonic crystals Wait.
As mentioned above, there are many options for the transmission structure in the terahertz frequency band, and it is necessary to select a suitable waveguide structure for different requirements.
At the same time, it is still necessary to find materials and structures for terahertz transmission lines with lower loss and dispersion.
3.3 Terahertz detection
Similar to the terahertz source, its detection methods can be divided into incoherent detection and coherent detection.
3.3.1 Non-coherent detection
Incoherent detection, that is, direct detection, refers to the use of a detector to directly convert the detection signal into a current or voltage signal, and obtain the amplitude information of the measured signal.
This detection method has a simple structure and a wide dynamic range, and is suitable for the detection of frequency bands such as millimeter waves, far-infrared rays, and visible light.
One of its remarkable advantages is that it can be detected by a large-scale detector array. However, due to the lack of its phase information, it is difficult to achieve ultra-high resolution.
The detectors used for direct detection are generally divided into uncooled detectors and cooled detectors. Uncooled detectors generally work at room temperature and have medium sensitivity and long response time.
Due to its low operating temperature, the cooled detector can obtain high sensitivity and fast response time.
3.3.2 Coherent detection
Different from incoherent detection, coherent detection usually adopts a superheterodyne structure similar to that in traditional communication systems, first transforming the terahertz signal to the lower microwave and millimeter wave frequency band, and then extracting the amplitude and phase of the signal in a traditional way.
Due to the frequency conversion method, the coherent detection system is more complicated, and key components such as mixers are needed. At the same time, higher requirements are put forward for the mixer and the terahertz local oscillator source, such as higher output power and lower noise. It is worth mentioning that, due to the detectable phase information, higher resolution can be obtained.
In addition, signal amplification can also be carried out, so that higher sensitivity can be obtained.
This technology is widely used in various high-resolution and high-sensitivity detection scenarios, such as deep space detection, etc.
Shi Shengcai’s team at the Purple Mountain Observatory in China has successfully developed superconducting mixers in the 500 GHz and 800 GHz frequency bands, and applied them in radio astronomy detection.
3.3.3 Geophone
Some commonly used detectors include:
- Schottky tube detector
Schottky tubes can not only be applied to direct detection, but also can be used as nonlinear devices in superheterodyne receivers.
When applied to direct detection, it has the advantages of high efficiency, low cost, and high integration; when applied to a mixing receiver system, high resolution and high sensitivity can be obtained. - Pyroelectric detectors
Pyroelectric detectors are devices designed by taking advantage of the heat-sensitivity effect between light radiation and matter, and have been widely used in far-infrared detection, and are now gradually used in the terahertz field. - Semiconductor bolometers
The bolometer generally uses a high-sensitivity thermistor to detect the corresponding resistance value change produced by the thermal radiation irradiated on the detector to obtain the power of the terahertz wave. Generally, the detectable frequency range is from tens of GHz to Dozens of THz. Classical bolometers contain heavily doped semiconductors. - Semiconductor thermionic bolometers
The high thermal conductivity and small heat capacity of the bolometer are realized by the interaction of the electrons in the semiconductor or superconductor with the lattice, which makes it suitable for the terahertz field. In the semiconductor thermionic bolometer, the non- Electrons in equilibrium are called hot electrons.
Unlike ordinary bolometers where the lattice absorbs power first and then transfers the energy to the free carriers, in this type of bolometer, the incident radiant energy is directly absorbed by the free carriers, so the lattice temperature remains unchanged. - Superconducting thermoelectron bolometers
In order to further improve the sensitivity of the semiconductor thermionic bolometer, the detector is realized by introducing a superconducting microbridge composed of NbN, NbTiN or Nb on the dielectric substrate.
Thermionic bolometers can be divided into two categories: one is the phonon-cooled thermionic bolometer; the other is the diffusion-cooled thermionic bolometer. - FET detector
Due to its low cost and high integration, CMOS technology has attracted extensive attention from researchers at home and abroad.
In recent years, the THz detection technology based on CMOS technology has made significant progress. In some early studies, field effect transistors have been applied to THz detection, and in some recent studies, detection arrays have been gradually developed and integrated in THz CMOS single chip. - Superconducting Transition Edge Sensor (TES)
The superconducting transition edge sensor uses electric current to heat the superconducting film to the superconducting transition temperature, which is a superconducting incoherent detection technology.
When detecting terahertz radiation, the superconducting film can absorb terahertz photons, causing the temperature to rise, and then the resistance to increase significantly.
This will reduce the current flowing through the superconducting film, thereby lowering the temperature and gradually returning to the original stable state.
This process is called electro-thermal feedback, in which a highly sensitive ammeter can be used to read the current change.
Currently the largest TES detector array application is the SCUBA2 detector array installed on the JCMT telescope in the United States.
3.4 Terahertz components
With the rapid development of terahertz technology, researchers from various countries have carried out a lot of research on terahertz components, including terahertz passive components, such as terahertz filters, terahertz antennas, terahertz couplers, and terahertz active devices. , such as terahertz frequency converter, terahertz frequency multiplier, etc.
3.4.1 Terahertz filter
The terahertz frequency band has an extremely wide physical bandwidth available.
In different applications, it is necessary to use passive structures such as filters to select the terahertz frequency.
Currently reported terahertz filters include frequency selective surface (FSS), microelectromechanical system (MEMS) filter, waveguide cavity filter, MMIC filter, CMOS and SiGe filter, etc.
In addition, there are some terahertz filters based on structures such as two-dimensional photonic crystals, metamaterials, and surface plasmons.
- The research on the FSS spatial filter in the low frequency band has been quite mature, while the
There are not many studies on FSS in the Hertzian band, and there are only few reports.
The main methods of designing FSS are multi-resonant unit, multi-layer structure method, perturbation method, genetic algorithm, etc. Frequency selective surface adopts periodic structure to realize frequency selection, which has been widely used in microwave, millimeter wave and quasi-optical systems. - The MEMS-based terahertz cavity filter is similar to the waveguide cavity filter, both of which are implemented with a cavity structure. The former uses a bulk silicon etching process to etch the filter pattern on the silicon, and then sputters gold on the silicon surface, etc. Realize the characteristics of the waveguide cavity, and the latter uses high-precision CNC machining technology to mill out the filter pattern directly on the metal.
In order to avoid expensive and difficult deep silicon etching, the high processing precision of MEMS can be used to etch periodic trench structures on the surface of silicon substrates, so that a terahertz low-pass filter with high selectivity can be realized. - The photonic crystal terahertz filter realizes the terahertz filter by introducing defects in the photonic crystal structure.
- Laser irradiating the quantum well structure can make electrons and holes combine to generate photons, and changing the carrier concentration can change the frequency of the plasma, so the optical properties of the quantum well structure can be controlled by the incident laser, and then design a quantum well structure based on
constructed terahertz filter. - The terahertz wavelength is relatively short, so it is possible to design passive structures such as terahertz filters using MMIC, CMOS, SiGe and other micro-nano processing technologies based on the traditional planar microwave and millimeter-wave circuit structures.
There have been few reports of research in this area.
3.4.2 Terahertz Antenna
With the deepening of research on terahertz technology, terahertz antennas have gradually become a research hotspot.
The terahertz frequency band has a higher operating frequency than the microwave and millimeter wave bands, and the corresponding wavelength is much shorter.
Due to the correlation between antenna size and wavelength, terahertz antenna has the natural advantage of small size, but it also brings challenges to processing. Similar to the antenna requirements for low-frequency communication, terahertz antennas are also divided into omnidirectional antennas, directional antennas, and multi-beam antenna arrays.
Common terahertz antennas include:
- The terahertz horn antenna has directional beam characteristics and high antenna gain, and has been widely studied and applied.
Due to the very small size of the antenna in the terahertz frequency band, the processing accuracy is extremely high, so the conical horn antenna produced by Rutherford Laboratory in the UK can work up to 2.5 THz. - The terahertz reflector antenna has the advantages of high gain, low sidelobe, narrow beam, etc. It is also an antenna form often used in terahertz technology, including single reflector antenna and double reflector antenna, which are generally widely used in radio astronomy telescope.
- The terahertz lens antenna adopts a dielectric lens, which has the characteristics of high gain and low sidelobe.
Due to its high integration and the ability to form a lens array, it has played an important role in promoting the development of terahertz imaging technology. - The terahertz planar antenna has a simple structure, is easy to integrate with other circuits, and is easier to process and lower in cost. It is a popular structural form.
- As a main method to generate broadband THz waves, photoconductive antennas have been extensively studied in the THz field.
Its role is to effectively generate high-power, high-energy, and high-efficiency terahertz waves, and its development trend is to continue to improve the power and efficiency of terahertz waves.
In addition, some new materials have also received attention in the design of terahertz antennas, such as carbon nanotube dipole antennas and on-chip terahertz 3D antennas.
3.4.3 Terahertz Mixer
In the superheterodyne terahertz system, the mixer is a core device, and its function is to move the terahertz signal down to the microwave and millimeter wave frequency band, so as to realize the acquisition, analysis and processing of the signal. Hertz imaging, atmospheric monitoring and other fields have an important impact.
At present, the mixing tubes that can be easily realized in the terahertz frequency range include superconductor-insulator-superconductor (SIS) mixing tubes, thermoelectron bolometer mixing tubes, and Schottky diodes.
The former two have higher requirements on the temperature of the working environment and require a low-temperature environment, but Schottky diodes do not have this limitation. The superconducting tunnel junction mixer is composed of a superconducting tunnel structure with approximately ideal switching characteristics. Therefore, it can provide higher frequency conversion efficiency and a certain frequency conversion gain, and at the same time it only needs a small local oscillator power, so the noise is low.
The commonly used Nb tunnel junction has good performance below 700 GHz, and if combined with high-gap superconducting materials NbN and NbTiN, the operating frequency can be extended to 1∼2 THz.
At present, the research on superconducting tunnel junction mixers gradually develops from unit design to multi-pixel design, especially the design of large-scale multi-pixel integrated receivers. In recent years, researchers at home and abroad have carried out a lot of research work on terahertz mixers.
3.4.4 Terahertz frequency multiplier
Similar to the terahertz mixer, the terahertz frequency multiplier is also a core device of the terahertz system.
Through frequency multipliers, not only can high-frequency terahertz signals be generated from low-frequency microwave and millimeter-wave signals, but also the frequency stability and signal quality of terahertz signals can be improved to a certain extent.
The main principle is to use nonlinear devices to generate twice or more times the output signal, so as to realize the function of signal frequency multiplication, which is an important means to obtain high-frequency terahertz signal sources at present.
Because in the terahertz frequency band, the parasitic parameters of semiconductor devices have a great influence on the circuit performance, it is necessary to analyze and model them carefully, and then complete the design of the frequency multiplier.
3.5 Terahertz applications
The development of terahertz technology has stimulated many meaningful applications and brought new developments in many fields. Due to space limitations, only a few applications in typical fields are listed below.
In terms of THz radio astronomy applications, because the cosmic background radiation contains abundant information in the THz spectrum, it makes THz radio astronomy an important means of astronomical observation.
By using terahertz waves to study the cosmic background radiation, we can understand more about the solar system we live in and the evolution of the universe. For example, by studying the terahertz frequency spectrum characteristics of interstellar molecular clouds, the origin of the universe can be explored; the spectral information scattered by atoms and molecules can be analyzed to study the formation of newborn galaxies in the universe, etc.
In terms of terahertz non-destructive testing, the photon energy of terahertz radiation is low, it will not cause damage to penetrating objects, and can pass through most dielectric substances. This characteristic of terahertz waves is very useful for detecting hidden defects in non-conductive materials or Special marking has a lot of room for development, generally known as non-destructive testing, such as testing oil paintings, spacecraft and semiconductor devices.
In terms of terahertz life science applications, because terahertz radiation waves are basically harmless to the human body, and water and other tissues have different absorption rates for terahertz waves, it can be widely used in local imaging of the human body and medical diagnosis of diseases , such as the detection of skin cancer and breast cancer.
The terahertz band contains a large amount of spectral information, and it will show different absorption and dispersion characteristics for different molecules, especially organic macromolecules, so it can be effectively used to determine molecular properties, and has broad application prospects in the field of life sciences. For example, the binding state of DNA, the characteristics of biological tissues and protein complexes are determined.
In terms of terahertz security applications, terahertz waves are penetrating and can effectively detect hidden objects, and can be used in national security-related fields, such as detection of hidden explosives, hidden guns, mailing of illegal drugs and It is used for fast security check at the airport, etc. Sun Xiaowei’s team at Shanghai Institute of Microsystems developed a 0.36 THz imaging system, University of Electronic Science and Technology Xue Fanyong’s team developed a 0.34 THz SAR imaging system.
In terms of terahertz high-speed communication, compared with the spectrum of the existing microwave and millimeter wave communication bands, the terahertz frequency band has massive spectrum resources, which can be used for ultra-wideband ultra-high-speed wireless communication, such as 100 Gbps or even higher.
4 Prospects for future research directions in the field of millimeter waves and terahertz
This section gives some suggestions on key research directions according to the current status and development trends of the millimeter wave and terahertz fields.
4.1 Millimeter wave field
- High power millimeter wave solid state source.
For 5G communication, space-space integrated communication, high-resolution radar and other application requirements, develop GaAs and GaN processes, increase the output power of millimeter-wave solid-state amplifiers, and explore the principles and implementation methods of high-efficiency power combining. - High power millimeter wave electric vacuum device.
The design and implementation of high-power amplifiers such as millimeter-wave traveling wave tube (TWT), gyrotron (Gyrotron), klystron (Klystron), and return wave tube (BWO) are focused on improving their reliability and life. - Millimeter wave III/V monolithic integrated circuits.
Research GaAs, InP and other III/V millimeter-wave monolithic integrated circuits, improve output power and noise performance indicators, increase circuit integration, and meet the application requirements of millimeter-wave technology in my country. - Millimeter wave silicon-based integrated circuits.
Silicon-based (such as CMOS, SiGe, etc.) millimeter-wave integrated circuits are worse than III/V monolithic integrated circuits in terms of power and noise performance, but the characteristics of high integration and low cost will make CMOS or SiGe integrated circuits In the future, millimeter wave applications will play an increasingly important role.
For applications such as 5G wireless communication, array imaging, and automotive anti-collision radar, the architecture and implementation methods of highly integrated, multi-channel millimeter-wave silicon-based SoCs are studied. - Development of millimeter wave measuring instruments.
At present, the field of millimeter wave test instruments in my country is basically monopolized by foreign companies such as Keysight and R&S, and test instruments are the basis for the development of various circuits and systems.
Therefore, it is necessary to strengthen the research and development of millimeter-wave measuring instruments, especially high-end millimeter-wave measuring instruments. - Millimeter wave application system.
Explore new principles, new architectures, and new implementation methods of millimeter-wave application systems, as well as their innovative applications in the fields of radar, guidance, communication, imaging, and automotive autonomous driving.
4.2 Terahertz field
- High power terahertz source.
High-power sources are crucial for terahertz long-distance imaging, high-penetration spectroscopy for probing the interior of matter, and terahertz communication.
But so far, there is no good solution for low-cost, small-volume, high-power terahertz sources, and continuous research is needed in order to obtain breakthroughs. In addition, terahertz traveling wave tubes, gyrotrons, klystrons, and klystrons should also be developed. High power amplifiers such as tubes and return wave tubes. - Terahertz signal detection.
Terahertz signal detection has made great progress in recent years, and the performance indicators of many technologies are close to their theoretical limits, but it is still difficult to meet the growing demand.
Therefore, it is urgent to explore new detection principles, discover new devices, and advance the research of large-scale terahertz detection arrays on this basis. - Terahertz solid-state devices and integrated circuits.
Develop III/V semiconductor technology, carry out research on component models and circuit design methods, realize terahertz devices and monolithic integrated circuits; develop silicon-based integrated circuit technology, carry out research on corresponding component models and circuit design methods, and realize terahertz Hertzian devices and system-on-chip. - Terahertz new materials and passive components.
Develop new high-precision processing technology and new terahertz materials, explore new working mechanisms of terahertz passive components, and study new low-cost, low-loss, and highly integrated terahertz passive components. - Ultra-fast terahertz communication.
For occasions where the future data transmission rate needs to be 100 Gbps or even higher, research on ultra-high-speed terahertz communication technology, including spectrum planning, channel model, system architecture and standards, etc. - Terahertz measurement technology and instruments.
With the maturity of terahertz wave generation and detection technology, the development of terahertz instruments in my country will also kick off.
Terahertz network analyzers, terahertz spectrometers, etc. play an important role in supporting my country’s research and development capabilities in the terahertz field. - Terahertz cross-application field.
At present, the main research field of terahertz technology is terahertz imaging, mainly focusing on biological imaging applications.
Terahertz waves can be used to detect some cells, DNA, etc. that are comparable to their wavelengths. To study the interaction between terahertz waves and various substances, in order to discover new physical properties, chemical changes, etc., provide a basis for research in the basic fields of biomedicine train of thought.
The development of new terahertz technology application fields plays an important role in promoting the overall development of terahertz technology.
In addition, promoting the application of existing terahertz systems to industrialization, and designing a commercial terahertz system that is easy to process, low cost, and high performance will bring revolutionary changes to the development of the country’s basic industries.
5 Conclusion
From the perspective of millimeter-wave and terahertz systems and applications, this paper systematically expounds the key technologies in millimeter-wave and terahertz systems, as well as the existing industrial applications, and looks forward to the future development of millimeter-wave and terahertz systems.
The field of millimeter wave and terahertz is a rapidly developing interdisciplinary subject, which has extremely important scientific research value and industrial application prospect. After decades of development, the research on millimeter wave and terahertz technology has achieved many important results.
However, it is still not mature enough in many research fields, and it is urgent to further in-depth development, and effectively enrich the application of these frequency bands, and finally promote the development of the national economy.