Research Progress of Terahertz Fiber
Terahertz, Optical fiber, Research progress, Review
Abstract
Terahertz(THz)band in electromagnetic spectrum attracts more and more attentions in recent years. Terahertz fiber is of great importance for the development of complex terahertz system. The wave-guiding mechanism, research progress, best materials, fabrication methods and application prospects of terahertz fiber are reviewed. The potential applications of terahertz fiber including short-distance data transmission, sensing and imaging are discussed.
Introduction
Terahertz is between microwave and visible light, covering to the frequency range of 0.1-10 THz corresponds to a wavelength between 30 µm and 3 mm.. With the advancement of terahertz technology, it has shown unique advantages and great application prospects in the fields of biology, medicine, electronic information, national defense and security. Therefore, the efficient transmission of terahertz waves has received great attention from researchers.
Traditional terahertz systems are mostly based on free space transmission, but terahertz transmission loss in free space is large and the transmission efficiency is low. Therefore, low-loss waveguide technology has become one of the key technologies for the new generation of terahertz systems.
In order to achieve efficient transmission of terahertz, several waveguide forms have been proposed in previous studies, including metal wires, subwavelength fibers, Bragg fibers, holey fibers, and antiresonant fibers. The metal wire was proposed in the early stage of the development of terahertz technology.
Although the metal wire has excellent transmission characteristics, the terahertz fiber based on the concept of special optical fiber can control the transmission characteristics of the waveguide more deeply, such as loss, birefringence, dispersion and transmission. Band, etc., has become a research hotspot in the field of terahertz. In this paper, the research on waveguide mechanism, materials, manufacturing process and application prospect of terahertz optical fiber is reviewed.
1 Waveguide mechanism of terahertz optical fiber
Similar to traditional optical fibers, the waveguide mechanism of terahertz optical fibers mainly includes: total internal reflection effect (Total Internal Reflection, TIR), modified total internal reflection effect (Modified Total Internal Reflection, mTIR), photonic bandgap effect (Photonic Bandgap, PBG), reflection Resonance effect (Anti-resonance) and topological channel effect, etc.
The schematic diagrams of various wave guiding mechanisms are shown in Figure 1, where the white area is air, and each wave guiding mechanism is briefly introduced as follows
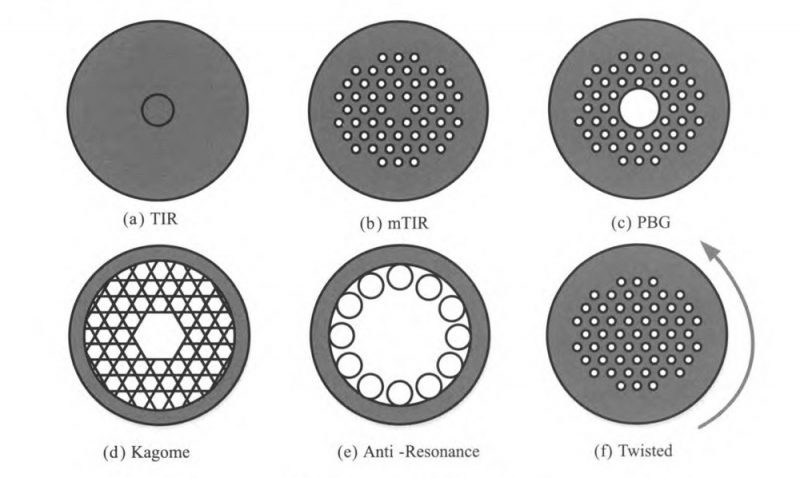
Fig. 1 The wave guiding mechanism of terahertz fiber
The TIR and mTIR effects are closely related to the refractive index difference between the fiber core and cladding, and the TIR effect allows terahertz waves to propagate in the high refractive index core, as shown in Figure 1 (a); the mTIR effect is formed when the cladding is a microstructure composed of background material and low refractive index material (usually air), as shown in Figure 1 (b), and the mTIR effect is essentially a TIR effect.
In microstructured fibers, the 2D microstructured cladding can form a “photonic bandgap” that prevents the transverse propagation of terahertz waves but allows them to propagate longitudinally in the core defects, as shown in Fig. 1 (c). In this case, terahertz waves can be guided by a “photonic bandgap” whose guided wavelength range depends on the refractive index difference between the cladding structure, cladding material, and periodic cladding material.
In particular, the photonic bandgap effect allows terahertz waves to propagate in the air core, which opens up more possibilities for applications in the fields of telecommunications and sensing.
However, not all hollow-core fibers with high-duty-cycle cladding microstructures support the PBG effect, as shown in Fig. 1(d), this lattice structure is called Kagome structure. The structure has a low cladding mode density, and the low density of the cladding mode reduces the probability of coupling between the core mode and the cladding mode, which provides the possibility to realize low-loss guidance in a wide spectral range. If the cladding microstructure with a high duty cycle is simplified as a circle of hollow circular tubes, the extremely low mode density supported by these circular tubes also reduces the coupling probability of the core mode and the cladding mode, forming an anti-resonance effect, such as Figure 1(e) shows.
At antiresonant wavelengths, constructive interference occurs within the core supporting THz transmission, confining THz waves to the air core. At the resonant wavelength, the terahertz wave is coupled into the circular tube to form a dissipative mode.
Furthermore, a new waveguiding mechanism is demonstrated in helically twisted coreless photonic crystal fibers. The helical twist structure provides a topological channel, so that there is no coupling between the mode close to the center of the fiber and the mode far away from the center of the fiber. Even a microstructured fiber without a core can still achieve low-limit loss transmission, as shown in Figure 1 (f ) shown. Terahertz optical fibers based on this waveguide mechanism can support the transmission of orbital angular momentum modes, and related research is still in its infancy.
2 Development status of terahertz optical fiber
The development of terahertz optical fibers is mainly based on the research of special optical fibers. The existing design schemes of terahertz optical fibers have explored almost all wave guiding mechanisms that have been applied in photonics. Terahertz optical fibers with different wave guiding mechanisms have their own advantages and disadvantages.
The solid-core terahertz fiber based on the TIR and mTIR waveguide mechanism is simple in design and easy to realize, but in this kind of fiber, the terahertz wave is transmitted in the solid core, which is greatly affected by the absorption loss of the material and the transmission loss is high. For low-loss terahertz fibers, the research direction is gradually shifting from the design of solid-core terahertz fibers to the design of hollow-core terahertz fibers.
Hollow-core terahertz fiber is mainly based on the PBG effect and anti-resonance effect. The terahertz fiber based on the PBG effect can achieve low dispersion transmission through the optimal design of the fiber cladding microstructure, but the structure of the terahertz fiber based on the PBG effect is relatively complicated. , usually the size of the optical fiber is large, the requirements for the manufacturing process are high and the transmission bandwidth is limited. Therefore, the anti-resonance terahertz fiber with simpler structure and wider transmission bandwidth has gradually become a research hotspot. This kind of fiber can achieve low-loss transmission and is easy to manufacture, but the structure of this kind of fiber is relatively poor and usually not a single mode transmission.
In the literature, terahertz fibers with different guiding mechanisms have been studied. According to the structure type of terahertz fibers, they can be divided into suspension core fibers, slot core fibers, holey fibers, hollow core fibers, anti-resonance hollow core fibers, Bragg Optical fiber and helically twisted optical fiber etc.
Table 1 lists the research status of some terahertz fibers, including the types of terahertz fibers, materials used, operating frequency, transmission loss, and research institutions.
Table 1 Research progress of terahertz optical fiber
Terahertz Fiber Type | Cross section of terahertz fiber | Terahertz Fiber Materials | Absorption coefficient | Working frequency | Type of Study | Research institute | Research time |
Suspended core fibe |
|
Cyclic Olefin Copolymer Resin (coc, commercially known as TOPAS) | 0.0898/cm | 0.95 THz | numerical simulation | Harbin Engineering University | 2018 |
Slotted core fiber |
|
TOPAS | 0.0103~0.0145/cm | 0.5~0.9 THz | numerical simulation | Boise State university | 2017 |
Porous core fiber |
|
TOPAS | 0.056/cm | 1 THz | numerical simulation | Rajshahi University | 2014 |
Porous core fiber |
|
TOPAS | 0.32/cm | 1 THz | numerical simulation | Metropolitan Institute of Technology | 2018 |
Anti-resonance fiber |
|
Cycloolefin polymer resin (COP, commercially known as Zeonex) | < 0.023/cm | 1 THz | numerical simulation | University of Adelaide | 2020 |
Bragg fiber with cobweb cladding |
|
High Density Polyethylene (HDPE) | 2.08×10^-6/cm | 3.3 THz | numerical simulation | Yanshan University | 2007 |
Porous core fiber |
|
Polymethyl Methacrylate (PMMA for short) | < 0.25/cm | < 0.8 THz | Experimental Study | University of Adelaide | 2009 |
Air-coremicro-structure fiber |
|
Polytetrafluoroethylene (PTFE, commercially known as Teflon, Teflon) | < 0.01/cm | 0.76 THz | Experimental Study | National Taiwan University | 2008 |
Suspended core fiber |
|
Zeonex | 0.133/cm | 0.3 THz | Experimental Study | University of Auckland and University of Sydney | 2011 |
Bragg fiber |
|
Teflon | < 0.05/cm | 0.5~2 THz | Experimental Study | Montreal Institute of Technology | 2011 |
3 Materials and manufacturing process of terahertz optical fiber
3.1 Materials of terahertz optical fiber
Suitable materials are crucial to realize low-loss THz fibers.
In addition to metals, studies have found that many polymer materials have low absorption loss in the terahertz band, such as cycloolefin polymer resin (COP, commercially known as Zeonex), cycloolefin copolymer resin (COC, commercially known as Topas ), Teflon (Teflon), high-density polyethylene (HDPE) and polymethyl methacrylate (Polymethyl Methacrylate, PMMA for short), etc., the transmission characteristics of some commonly used materials in the terahertz band are shown in Figure 2.
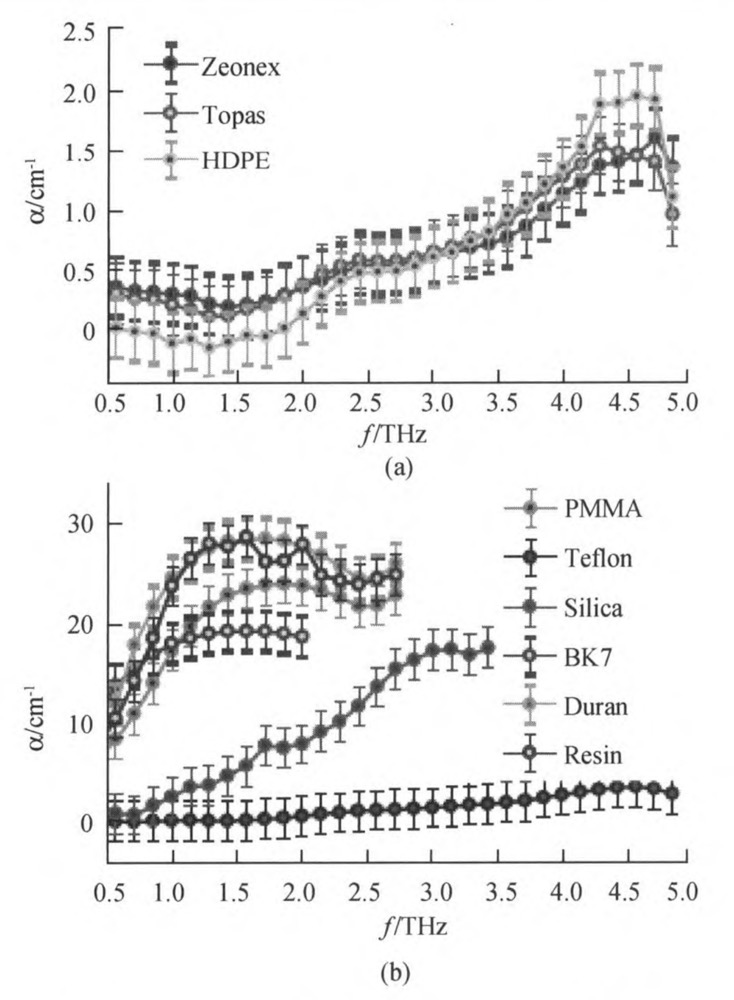
Fig. 2 Transmission characteristics of some common materials in the terahertz band
It can be seen from the figure that among the polymers Zeonex, Topas and HDPE have similar characteristics, and the absorption coefficient between 0.5 and 2 THz is less than 0.5/cm. However, Zeonex and Topas have lower losses than HDPE at higher frequency bands. Among the glasses, silica (Silica), borosilicate crown glass (BK7), Duran glass (Duran) show lower absorption loss.
Among 3D printing materials, UV-resin can provide higher resolution, but has higher loss compared with Zeonex and Topas, and its absorption coefficient at 1 THz is about 25/cm. From the terahertz transmission characteristics of polymers, glasses and UV resins, it is known that the absorption coefficient of polymers is lower than that of other materials, and Zeonex and Topas in polymers exhibit lower absorption characteristics.
3.2 Fabrication process of terahertz optical fiber
When developing a new type of terahertz optical fiber, in addition to selecting the appropriate material, it is also necessary to select the appropriate manufacturing process. The preparation process of terahertz optical fiber borrows from the production method of microstructure optical fiber. Generally speaking, terahertz optical fiber preform can be manufactured by drilling, stacking, extrusion, casting and 3D printing, and then the prefabricated The rod is drawn into an optical fiber.
This section will briefly introduce these fabrication methods.
3.2.1 Drilling method
Drilling is the most commonly used technique in the manufacture of polymer optical fiber preforms.
This method is mainly used to prepare optical fiber preforms with round holes, and computer numerical control drilling machines are usually used for preform processing.
During the drilling process, the drilling parameters such as cutting speed, spindle speed and depth of cut need to be optimized to avoid the rough surface of the air hole or the melting of the preform material due to excessive drilling temperature.
The maximum length of the preform and the size of the air holes that can be manufactured by this method are limited by the length and diameter of the drill bit in actual production.
3.2.2 Stacking method
The stacking method is another commonly used preform fabrication method. The method consists of manually stacking a large number of polymer capillaries and holding them together with the polymer sheath through this square tube to form the final preform. Through this method, complex structures that cannot be realized by various drilling methods can be realized, and the air pressure control method is used to form the drawing during the drawing process.
The stacking method is a common method for preparing high-duty ratio hollow-core optical fibers and holey optical fiber preforms. Although manual stacking is time-consuming and laborious for mass production, this method can overcome the limitation of the drilling method on the length of the preform.
3.2.3 Casting method
The casting method is also a common technique for the manufacture of polymer optical fiber preforms.
This method places the necessary chemicals into a mold, and after polymerization is complete, the mold is removed to yield the desired polymer preform. This molding technology can realize the production of preform rods of different shapes and sizes by changing the structure and arrangement of the mold.
3.2.4 Extrusion method
The extrusion method can directly prepare the billet into a polymer preform or a polymer optical fiber. The geometry of the exit of the extrusion die determines the cross-section of the fiber.
The key steps in extrusion molding are the design and processing of the mold. Each extrusion requires die replacement or cleaning, making the fabrication expensive and time-consuming, but extrusion can be used to create a wide variety of fiber structures.
3.2.5 3D printing method
3D printing is a well-established and relatively easy method of fabricating terahertz optical fibers. Due to the longer wavelength of terahertz waves, the microstructure size of terahertz fibers is comparable to the resolution of 3D printers, so terahertz fibers can be printed directly.
The short manufacturing cycle of 3D printing technology allows rapid prototyping of fiber designs, however, in order to improve the surface roughness of 3D printed terahertz fibers, a compromise must be made in the choice of fiber materials.
4 Application prospect of terahertz optical fiber
Terahertz optical fibers are still in the development stage, and the current main application directions include short-distance data transmission, sensing and imaging.
For transmission and communication applications, terahertz fibers must have properties such as low loss and low dispersion.
Although there is no optical fiber material with negligible loss, the transmission loss of optical fiber can be further reduced by optimizing the optical fiber structure design. In sensing applications, terahertz fibers can increase the interaction between terahertz waves and analytes, thereby improving the sensitivity of sensors.
At present, hollow-core terahertz optical fibers have been used in refractive index analysis and material concentration sensing, and terahertz optical fibers can be used as probes in imaging systems to transmit and collect sensing signals.
4.1 Transmission and communication
The main function of terahertz fiber is to reduce the influence of external environment on terahertz transmission. In recent years, a lot of research work has been done on the development of low-loss, low-dispersion terahertz fibers to improve terahertz transmission characteristics. In 2018, Gautam et al. proposed a graded rectangular core terahertz fiber for terahertz communication, which achieved a low absorption coefficient of 0.2/cm.
In 2019, Sen et al proposed a suspension-core graded-index fiber. Numerical studies show that by introducing a graded index into the porous core, a group velocity dispersion of 0.14±0.07 ps/(THz cm) can be obtained between 0.71 and 0.95 THz, and a group velocity dispersion of 0.0152±0.0004 can be achieved between 0.8 and 1 THz ps/(THz cm) intermodal dispersion.
In addition, helically twisted antiresonant fibers supporting orbital angular momentum (OAM) mode transmission are expected to be used in quantum communication and other fields. Although these designs are mostly studied numerically, they can be applied practically through the fabrication methods presented in the previous section.
4.2 Sensing
Similar to traditional optical fibers, terahertz optical fibers can also be used to detect liquids, gases, and biochemical substances. In 2016, You et al. built a Teflon tube-based NH4Cl detection system with a tube length of about 30 cm. The actual system is shown in Figure 3. The detection sensitivity of the system is 13.63 μg, and the corresponding measurement accuracy is 165 nmol/mm.
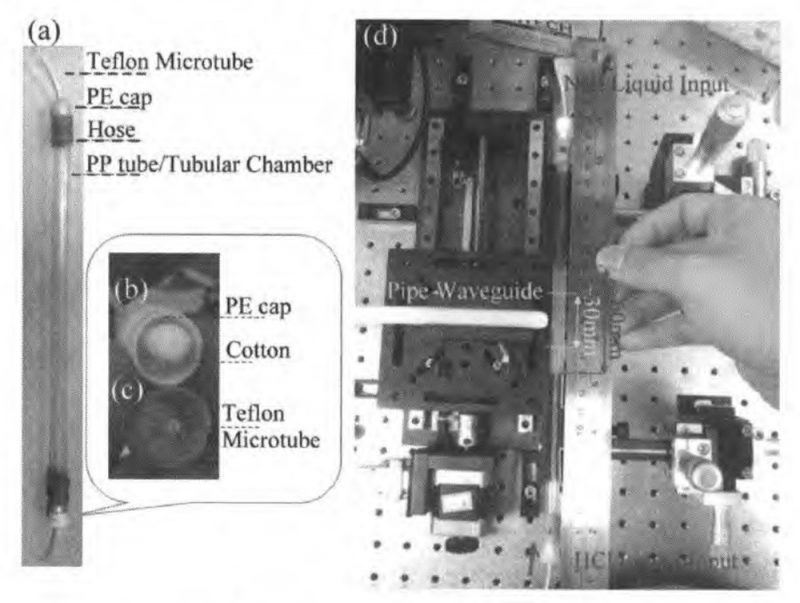
Fig. 3 NH4Cl detection port system based on Teflon tube
In addition, the research group also proposed a terahertz refractive index sensor based on a dielectric tube waveguide with a measurement sensitivity of 22.2GHz/RIU.
In 2017, Li et al. proposed a surface sensor based on terahertz Bragg fiber, The optical fiber is made by 3D printing. By introducing defects in the first layer, the thickness of α-lactose-hydrate is measured by coherent detection. The measurement sensitivity of the sensor is 0.1 GHz/μm.
In 2018, Islam et al proposed a suspension-core terahertz optical fiber based on Zeonex material for the detection of cyanide.
In 2019, Qin et al. proposed a TOPAS-based porous terahertz fiber for the detection of HCN, and the detection sensitivity is expected to reach 2×10-6. Numerical studies have shown that terahertz optical fibers have good application prospects in biological sensing and chemical reagent sensing, but further experimental verification is still needed in terms of applications.
4.3 Terahertz fiber optic imaging
Terahertz imaging systems are ideal for non-destructive testing, concealed object inspection, and biological detection. In 2008, Lu et al realized a terahertz imaging scanner using polyethylene subwavelength fiber as a probe.
The scanner can realize 2D large-scale scanning through the bending of the optical fiber, and the imaging signal-to-noise ratio of the scanner is better than 30dB. The image obtained by the scanner is shown in Figure 4.
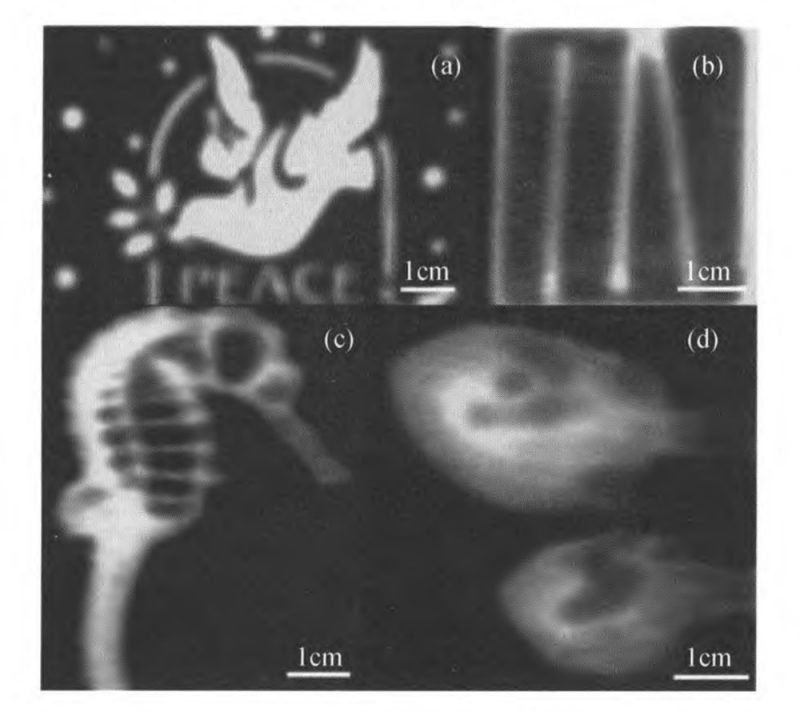
Fig. 4 Terahertz fiber imaging results
In 2014, Lu et al. used a terahertz optical fiber made of Zeonex material as a probe to realize a terahertz hyperspectral imager. The imager used three transmission windows between 0.1 and 1.2 THz, and the imaging resolution was about 1 mm. In addition, Chen et al. realized the diagnosis of breast cancer using a terahertz near-field imager. Based on the same principle, the same system is also used for the diagnosis of liver cancer. In the foreseeable future, terahertz fiber-based imagers will play a major role in medicine, security and other fields.
5 Conclusion
This paper reviews the research progress of terahertz optical fibers, including the main wave guiding mechanism, commonly used materials and preparation processes of terahertz optical fibers, and the application prospects of terahertz optical fibers.
This paper summarizes the wave guiding mechanism of terahertz optical fiber, mainly including total internal reflection effect, modified total internal reflection effect, photonic band gap effect and anti-resonance effect.
The structural types of terahertz fibers mainly include suspension core fibers, slot core fibers, holey fibers, Bragg fibers, hollow-core photonic bandgap fibers, and anti-resonance hollow-core fibers.
Each type of fiber has its own advantages and disadvantages, requiring a trade-off between low loss, bandwidth, and fabrication complexity. Studies on polymers have shown that Zeonex and Topas have lower absorption loss and are suitable as materials for low-loss terahertz optical fibers.
The various fabrication methods discussed in this paper provide the possibility for the preparation of terahertz optical fibers. Although the transmission loss of terahertz optical fiber is still relatively large at present, it shows great application prospects in the fields of short-distance data transmission, gas, chemical sensing and imaging. It will promote the further development of the above fields.